Summary
Most carbonates are extremely susceptible to mineralogical and textural change, cementation and dissolution. These alterations can occur at any time from initial depostion to deep burial. Most diagenetic changes affect porosity and thus must be considered in the exploration for carbonates .
Diagenesis of carbonates begins at deposition and continues during burial and uplift (left figure). When carbonates are brought into contact with waters of varying chemical composition, they have a great susceptibility to mineralogical and textural change, cementation and dissolution. Thus, diagenesis is greatest near the sediment surface and during shallow burial, where most variation in mineralogic composition of the carbonates and ground waters occurs (figure below). As carbonates are buried more deeply, they are commonly in equilibrium with the adjacent subsurface waters; nevertheless, significant diagenetic changes can occur.
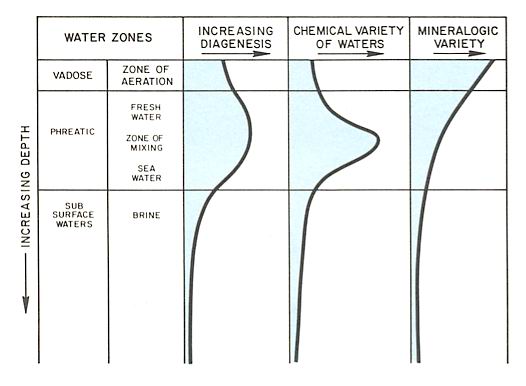
Our understanding of deep subsurface diagenesis is incomplete, but we do know that dolomitization, pore-fill cementation and pressure solution occur at depth and may be extremely important. During uplift, fracturing, additional cementation and leaching may occur. (More illustrations of carbonate diagenesis can be reached at a galllery of carbonate diagenesis that can be reached by clicking on the highlighted text).
Shape and geochemistry of cement crystals and zones are important to their interpretation in ancient deposits, since the mineralogy has changed and is probably in equilibrium with the present subsurface fluids. For instance, in marine waters aragonite and magnesium calcite form fibers, blades and micrite which can be recognized despite later replacement by calcite. Fresh waters and deep subsurface brines generally precipitate calcite cements as silt-sized or larger rhombic crystals or micrite. Marine waters with high salinities or fresh waters with high magnesium to calcite ratios may form dolomites.
The carbonate cements
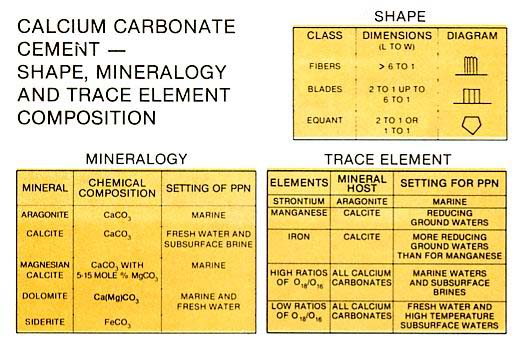
carbonate cements contain trace elements. Those that are of immediate interest to the carbonate geologist are strontium, manganese and iron because they provide data that can be used to interpret cement origin. Strontium substitutes for calcium in the aragonite lattice, whereas when the waters in contact with the cement are reducing, manganese and iron substitute for calcium in the calcite lattice. Manganese and iron are especially important because they occur in ancient limestones and dolomites. Trace element composition of carbonates is studied by staining of Rock slabs or thin sections, chemical analysis, cathodoluminescence, and EDAX or microprobe (see Scholle, 1978, p. 225-233 for a simplified explanation of these techniques). Stable isotope composition of carbonates can be used, like trace elements, to unravel carbonate diagenetic history. For instance, the two most common isotopes of oxygen, 180 and 160, have different compositional ratios depending on the diagenetic environment. The lighter 160 is more common in cements precipitated from fresh water and/or subsurface waters with elevated temperatures and is rarer in cements associated with marine waters. Similarly the common isotopes of carbon, 13C and 12C, show much the same relationship.
Lighter carbon is associated with fresh water and deeper burial. Some of the light carbon may be derived from organic carbon associated with migrating hydrocarbons. In cases where oxygen and carbon isotopes have been systematically analyzed through a cement, they lighten from the base of a crystal to its margins.
The setting and timing of diagenesis is interpreted by the petrography and geochemistry of cement crystals along with their distribution within the Rock (left figure). For instance, cement crusts form in both marine and fresh-water phreatic environments. The marine crusts are commonly isopachous fringes of aragonite fibers or magnesium calcite blades. Unfortunately the latter may be confused with fringes of calcite blades that form in fresh waters. Irregular crusts are associated with poor permeabilities or low rates of calcium carbonate precipitation, and isopachous crusts are associated with good permeabilities. Cements precipitated from fresh waters in the vadose zone typically have a patchy distribution, reflecting the occurrence of both air and water in the pore space. Where pores are incompletely filled, the cement occurs only at grain contacts and crystals have curved meniscus faces. Late-stage, deep burial cement crystals are also unique. They commonly are large crystals filling more than one interparticle pore. They can be confused with early-formed epitaxial overgrowths on echinoderm plates in a Rock that contains echinoderm debris.
Diagenetic Setting
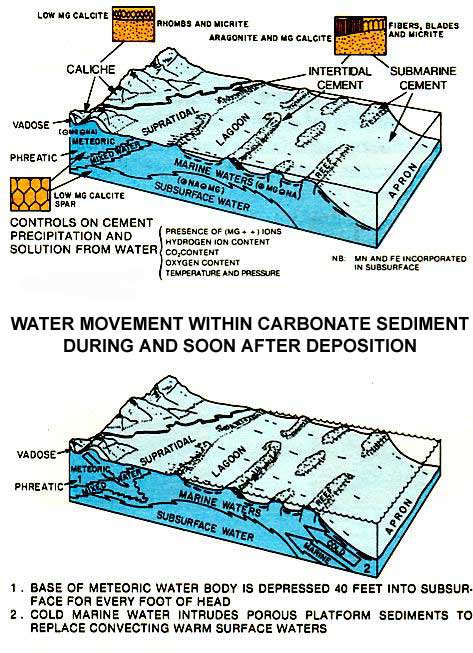
The diagenesis of carbonates can take place in many settings: the marine environment during deposition of the sediment, near the sediment surface where fresh waters penetrate the sediments, or in brines of the deeper subsurface (figure above). The diagenetic setting can be reasonably interpreted with detailed study. In this way the porosity evolution of a carbonate sequence can be unraveled to more accurately predict reservoir porosity trends.
Marine Setting
Marine cements form in a broad spectrum of environments, extending from the deep sea to beaches (left figure). Deep sea cements, commonly mammilated or isopachous layers of magnesium calcite and aragonite, produce hardgrounds where there is good bottom current movement. The flanks of carbonate platforms and the margins of submarine channels and canyons are such sites. Other deep-sea cementation may also occur in areas of negligible sedimentation and may be associated with volcanics or with elevated salinities in enclosed basins.
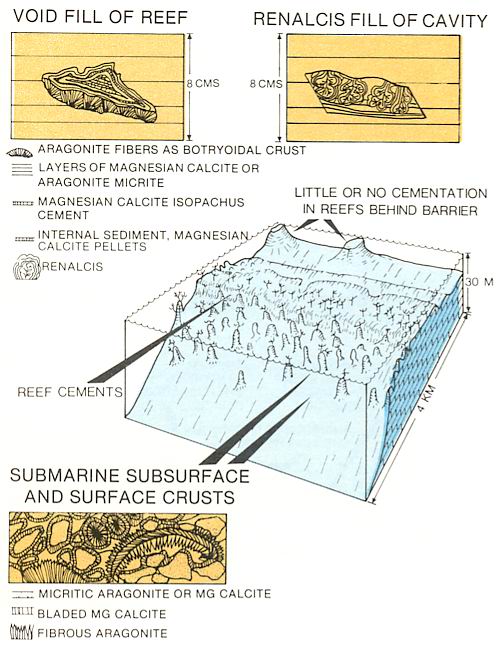
Cementation is common in shallow water where the reef margin is one of the better-documented examples (above figure). Reef boundstones are commonly tight, thus dissolution by fresh waters or fracturing are important processes in creating reservoir quality porosity. Reef cementation usually occurs as bladed or pelleted micritic magnesian calcite or fibrous aragonite cements. The fabric of the cement is controlled by access to circulating water. Voids with greater permeability may be filled by isopachous or equant cements whereas the more confined voids show irregular fibrous crystals. The zone of active cementation in reefs appears to be confined to the outer few feet, where circulation of waters being pumped through the reef is at its greatest. Evidence of this localization of cementation has been recognized in the reefs from Belize, which are cemented only on their seaward margin.
Eniwetok atoll (left figure) provides a good example of changing cementation patterns through time. The initial cements in reef debris are magnesium calcite and aragonite marine cements. The marine cements were followed by a fresh water calcite cement which in turn may have marine sediment perched on it. The cement sequence is a response to the different waters which came into contact with the sediments during a sea level lowering and reflooding.
Syndepositional cementation of carbonate sediments also occurs on the shallow platform or shelf immediately behind a break in slope. Cementation occurs during the formation of oolites, grapestones, and hardened pellets. For instance, the oolites which occur in high energy zones are formed by the precipitation of calcium carbonate around a nucleus. Grapestones, found to the lee of oolite shoals in a quieter water setting, are formed by the precipitation of carbonate cement at points of contact between sand-sized grains and by partial disaggregation of cemented crusts by storms. Further evidence of cementation is the hardening of pellets on open shelf seabottoms, whereas in protected water leeward of the hardened pellet zone on the Great Bahama Bank, lime mud pellets remain uncemented.
Submarine cemented crusts that are common to platform settings are formed during deposition. In the Arabian Gulf, extensive cementation in crusts causes the crust to expand and override itself forming compressional ridges or submarine tepees (figure above). These mark the margins of saucer-like expansion megapolygons that form during the cementation and expansion of these submarine surfaces. Submarine crusts or hardgrounds can be recognized in the Rock record by the presence of multiple generations of borings which cut through both sediment and cement.
Marginal Marine Setting
Cementation in nearshore zones is as variable as the ground water of these areas (left figure). Surface and subsurface crusts that form at the shoreface of the beach and within the shallow sediments immediately offshore are largely marine in origin but may have a freshwater overprint. The hardened layers on the shoreface of the beach are termed beachRock. Crusts on supra-tidal flats generally show evidence of desiccation and expansion. Ridges, partly formed by the cementation of sediment fill of desiccation andexpansion cracks, outline polygonal saucers with depressed centers (figure below). These peritidal tepee structures are similar in appearance to marine tepees. They can be differentiated on the basis of the types of cements and the associated sediments. Travertines, pisolites, boxwork structure and soil horizons are commonly associated with the pertidal tepees.
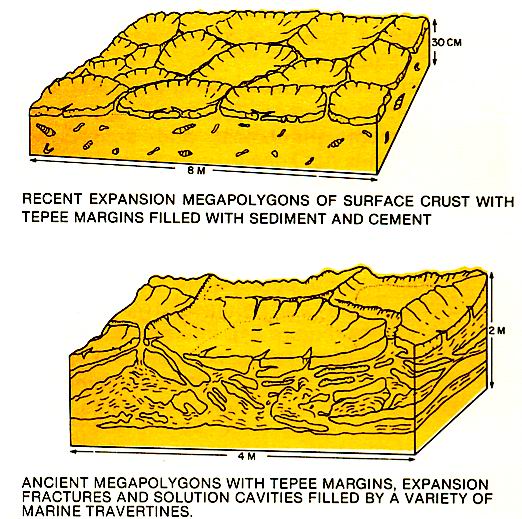
In supratidal flats of arid areas, important mineralogical changes commonly occur.
Aragonite sediments become dolomitized and evaporites are emplaced in the carbonates (left figure). Landward, gypsum followed by anhydrite and halite may be precipitated. The gypsum may form individual displacive crystal laths or layers of mush, whereas anhydrite occurs in contorted layers or as nodules. Where the sulphates form in standing bodies of water, they form horizontal layers that parallel the sediment-water interface. The occurrence of evaporites at the updip end of a carbonate shelf or platform is important because the evaporites often form the updip seals to reservoirs developed in dolomitized shelf carbonates .
The association of dolomite and ancient tidal flat deposits is common. The exact mechanism for magnesium enrichment and subsequent dolomitization is not known, but several theories have been proposed (left figure). Freshwater mixing with marine waters that are washed onto tidal flats and evaporated are likely mechanisms in the tidal flat environment. However it is formed, the dolomite has the potential for creating reservoir quality porosity and permeability in originally tight limestones (figure below). Intercrystalline porosity in dolomites is responsible for many Paleozoic reservoirs, a good example being the Mississippian Little Knife field carbonates .
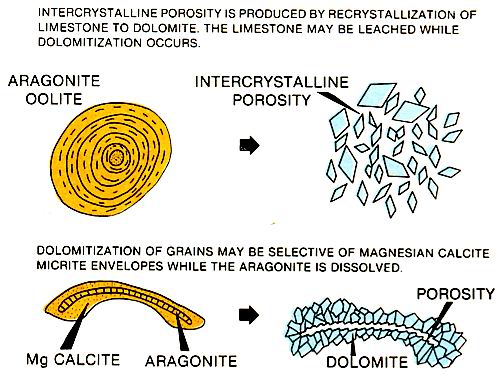
Fresh Water Setting
As marine carbonates come into contact with fresh water, significant diagenesis occurs. The formation of secondary porosity by dissolution is the most important process in reservoir development. During deposition fresh water may interact with marine sediments in the supratidal zone and sometimes in the intertidal zone. If the relief or size of the fresh water recharge area is sufficiently great, a fresh water lens may extend well beyond the land mass into marine sediments. Maximum diagenesis associated with fresh water occurs at the Rock-air interface and in the zone of mixing between fresh water and seawater or fresh water and brines (figure below).
Cements include calcite and possibly dolomite. calcites precipitated in the zone of aeration above the water table, ie., vadose zone, are blocky and fibrous-needle calcites. Where pores are incompletely filled, the cement is confined to points of contact between grains or to the undersides of grains. This asymmetry in the cements does not occur below the air-water interface. Below the water table, calcite cements commonly form rims of bladed to equant crystals that completely encircle the grains.
The diagenesis associated with fresh water includes the inversion of aragonite to calcite, the loss of magnesium from magnesium calcite and dolomitization (left figure). Aragonite and magnesium calcite, both minerals common to the marine environments, are unstable in magnesium-deficient waters regardless of whether these waters are fresh, brackish or saline. These minerals change to calcite by leaching and reprecipitation. In some cases the original mineral fabrics are completely preserved and in others they are completely destroyed. The aragonite to calcite transformation may cause partial plugging of the existing porosity by precipitation of calcite cement. In carbonates that are tightly cemented a source of the cement other than the transformation of aragonite to calcite is required. Most probably, the additional carbonate is derived from the weathering and dissolution of adjacent carbonates .
Subsurface Setting
Cements formed in subsurface brines are commonly equant iron-rich calcite spar with crystals that have flat faces. If magnesium to calcium ratios are high, particularly in mixing zones of brines with different compositions, dolomitization may locally be important. Although the deep burial diagenetic realm is not as well understood as near-surface conditions, it is apparent that pressure solution compaction, cementation, and dolomitization may occur. The subsurface fluids responsible for the diagenesis can be derived from a variety of sources (figure above), but a likely source is the down-dip basinal shales and fine carbonates that expel fluids as they are compacted during burial.
Compaction in carbonates can cause significant restructuring within the Rock. In grain-rich Rocks, the grains may be flattened, broken or dissolved at grain contacts (right figure). Pre-burial cements may be similarly affected, resulting in a change of the porosity and permeability patterns in the Rock. Stylolites and other pressure solution features are commonly formed during burial or tectonic stress of mudstones and wackestones. The formation of such features is important because vertical permeability patterns are created and pore fluids are displaced.
porosity
Because of the broad-spectrum of diagenesis that affects carbonate Rocks, the final porosity in carbonates may or may not be related to depositional environment. Unlike other lithologies, the original primary porosity in carbonates may be totally destroyed during diagenesis and significant new secondary porosity may be created. The types of porosities encountered are quite varied (figure below). Interparticle, intraparticle, growth-framework, shelter and fenestral porosities are depositional porosities. porosity formed during diagenesis may be moldic, channel, inter-crystalline, fracture or vuggy porosity. (More illustrations of porosity can be reached at a galllery of carbonate porosity that can be reached by clicking on the highlighted text).
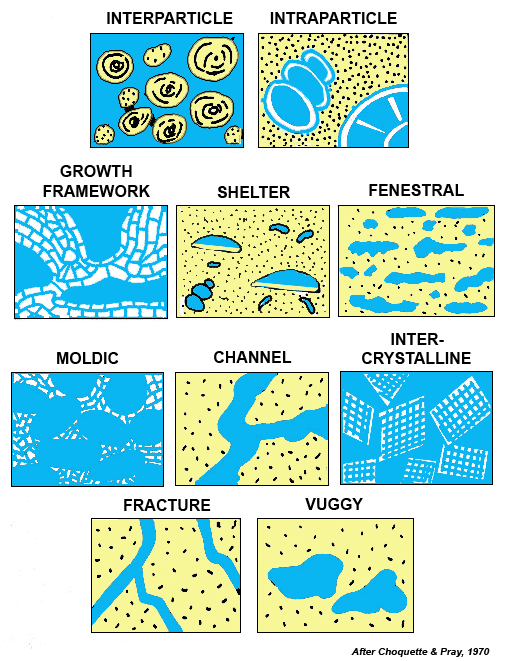
Depositional porosity is a function of Rock texture, grain sorting and shape. Sorting and shape in turn are related to bottom agitation at the depositional site. Where currents and waves are particularly active, lime mud is winnowed from carbonate sands. In contrast, lime muds tend to collect in less agitated environments or where trapped by organisms, and after the sediment dewaters little or no porosity is retained. Many of the world's larger carbonate reservoirs have porosities that are largely depositional in origin. Examples include the Jurassic Smackover limestones in southern Arkansas and northern Louisiana, the lower Cretaceous Sligo limestones of Black Lake in Louisiana, the Devonian Leduc limestones at Redwater in Alberta and the Mississippian Madison limestones in North Dakota and Saskatchewan.
The relationship between porosity and diagenesis is complex and variable. The major diagenetic processes affecting porosity are dissolution, cementation and dolomitization. Each process requires a permeable host Rock and a mechanism to flush chemically active waters through the Rock. The water movement is controlled regionally by the hydrostatic head, structure and Rock fabric. Dissolution creates and enhances porosity. Commonly, however, dissolution of carbonate grains is accompanied by calcite cementation in adjacent primary pores. The end product in such a case is tightly cemented carbonate sand with well-developed moldic porosity and low associated permeability. Cementation is an extremely important diagenetic process because it reduces porosity. The degree of cementation varies from thin cement coatings around the grains that partially fill the pores and alter permeability patterns to calcite spar that completely fill the pores.
Dolomitization may reduce, redistribute, preserve or create porosity. In a few carbonate reservoirs, as in the Jurassic Arab limestones of Ghawar field in Saudi Arabia, replacement dolomite crystals extend into adjacent pores thereby reducing the primary porosity. In many dolomitized reservoirs such as the Jurassic Smackover formation of Alabama and the Leduc reef carbonates in Alberta, porosity and permeability were redistributed during dolomitization and associated leaching. Early dolomitization may preserve porosity by creating a rigid framework that inhibits compaction. In still other cases dolomitization in lime muds may enhance porosity, because dolomites are denser and so consequently take up less volume than the original calcite. porosity that was formed during dolomitization is common in the Mission Canyon and Red River formations of the Williston basin.
porosity Preservation
The existence of carbonate reservoirs with preserved primary porosities commonly indicates incomplete cementation in near-surface environments.
Perhaps burial was rapid and the carbonates underwent only brief diagenesis in near-surface settings (left figure). Another possibility is that evaporites, shales, red beds or dense micrites formed a protective impermeableseal over the porous carbonates and prevented fresh-water flushing. The same near-surface waters that are responsible for cementation of carbonate sequences may also produce secondary porosity during dissolution. If the right balance is met in the near surface between precipitation and dissolution, an attractive reservoir Rock with good porosity and associated permeability can form.
Deep burial processes such as cementation and grain to grain interpenetration by physical compaction or pressure solution may be very important in porosity reduction. Thus, carbonate porosity preserved through shallow burial may or may not be preserved in the deeper subsurface. Accordingly, predictions of porosity in ancient carbonates should consider both the depositional environment where primary porosity is generated and the diagenetic environments where porosity can be enhanced, preserved or occluded.
USEFUL REFERENCES
Diagenesis
Bathurst, R. G. C., 1971, carbonate Sediments and their Diagenesis: Elsevier, New York, pp. 321-543.
Milliman, J. D., 1974, Marine carbonates: Springer-Verlag, New York, pp. 270-313.
Scholle, P. A., 1978, A Color Illustrated Guide to carbonate Rock Constituents, Textures, Cements, and Porositites: AAPG Memoir 27, Tulsa, pp. 129-168.
Longman, M. W., 1980, carbonate Diagenetic Textures from Nearsurface Diagenetic Environments: AAPG Bull., V. 64, pp. 461-487.
porosity
Choquette, P.W. and Pray, L.C. (1970). Geologic nomenclature and classification of porosity in sedimentary carbonates, AAPG Bulletin, v.54, No. 2, p.207-250.
Lucia, F. J., Jennings, J. W., Jr., Rahnis, M. A., and Meyer, F. O., 2001, Permeability and Rock fabric from wireline logs, Arab D reservoir, Ghawar field, Saudi Arabia: GeoArabia, v. 6, no. 4,
p. 619–646.
Lucia, F. J., 1995, Rock fabric/petrophysical classification of carbonate pore space for reservoir characterization: American Association of Petroleum Geologists Bulletin, v. 79, no. 9, p. 1275–1300.
Lucia, F. J., 1995, Chapter 14, Lower Paleozoic cavern development, collapse, and dolomitization, Franklin Mountains, El Paso, Texas, in Budd, D. A., Saller, A. H., and Harris, P. M., eds., Unconformities and porosity in carbonate strata: American Association of Petroleum Geologists, AAPG Memoir 63, p. 279–300.
Lucia, F. J., 1999, carbonate reservoir characterization: New York, Springer Verlag, 226 p.
Lucia, F. J., and Major, R. P., 1994, porosity evolution through hypersaline reflux dolomitization, in Purser, B. H., Tucker, M. E., and Zenger, D. H., eds., dolomites, a volume in honour of Dolomieu: International Association of Sedimentologists, Special Publication No. 21, p. 325–341.
Lucia, F. J., and Conti, R. D., 1987, Rock fabric, permeability, and log relationships in an upward shoaling, vuggy carbonate sequence: The University of Texas at Austin, Bureau of Economic Geology Geological Circular 87 5, 22 p.