Introduction to deepwater system sediments and their classification
Miall’s (1999) statement that "At the very least, classifications represent way stations on the road to perfect understanding; at best, they offer a common language for description and interpretation" is a mantra to the sections below that describe and classify deepwater sediments and the models that explain their deposition.
Dott and Bourgeois (1983) dismayed at the explosion of fluvial models, argued that this proliferation “defeats the whole purpose of the conceptual model by encouraging excessive pigeonholing, which obscures rather than reveals whatever unity may exist among the variants’’. The same could be said of deepwater sediments. Though deepwater sedimentary classifications are changing rapidly (Shanmugam, 2000) and proliferating, this web site argues that a consensus is developing amid the various schemes. The intent is that these models be used as a guide to the interpretation and the prediction of the continuity of each unique set of deepwater sedimentary Rocks and sediments. The solution is to consider the origins of the deepwater sediment you are examining and describe them carefully and in detail. This should be from the perspective of process driven models, with classifications being used as needed and modified to meet the specifics of the situation! Remember to always define your terms!
Deepwater Sedimentary end members of subaqueous density flows; namely non-cohesive density flow sediments (including turbidites), mass transport debrites (MTD) and pelagic drape and/or condensed sequences.
Data sources for the interpretation of deepwater sediments collectively include:-
- Outcrops
- Cores
- Well Logs
- Seismic Data
- Flume and Computer Simulation Experiments
The common characteristic of early classifications of deepwater systems was tied to the occurrence of beds of sandstone in which the sediment grain size fines upward. beds with this texture became known as deepwater “turbidites” (Kuenen, 1957). This was based on recognition that deposition from a waning turbidity current had a matching decrease in grain size. Later this vertical change in fabric became known as the “Bouma sequence” (Shanmugam, 2000). It is now recognized that while not every deepwater sedimentary cycle has the fabric of the Bouma sequence turbidite (Shanmugam, 2000), process driven interrelationships exist between most deepwater sediment facies and their associated geometries. Many excellent examples of these process driven interrelationships exist. One example that has caught the attention of stratigraphers is the Carboniferous Ross Sandstone of Co. Clare, Ireland. Pyles and Bouroullec (2006) recognize that this section exposes an ascending order of interrelated mudstone sheets and slumps, linked debrites and mudstone sheets, and non-cohesive density flow sandy turbidite lobes.
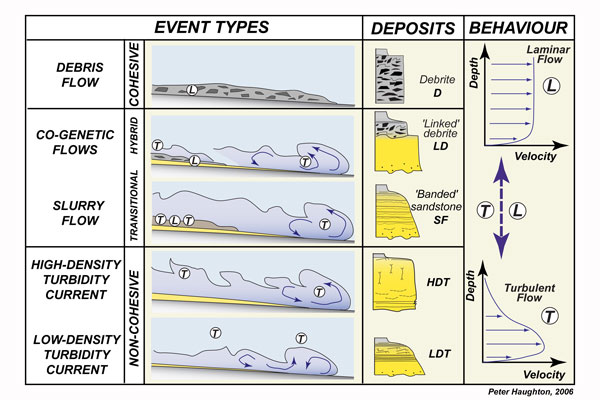
The above illustration by Peter Haughton demonstrates the evolving interrelationship between the various sub-aqueous density flows and their transportation of sediment. The diagram demonstrates how cohesive flows change to non cohesive flows while passing through transitional hybrid flows. The sediment from these different flows are co-genetic and slurry flows accumulates as a mix both cohesive and non-cohesive material. To conclude the sediment transportation evolves from cohesive flows to non cohesive high to low density turbidity currents. The above diagram simplifies the classification of Mulder & Alexander (2001) below.
The classification of Mulder & Alexander (2001), seen in the illustration above, captures the interrelationships of subaqueous density flows and subdivides them into cohesive flows and frictional flows. This is "based on the cohesivity of the particles, flow duration, sediment concentration and particle-support mechanism".
Cohesive flows have matrix strength and are divided by grain size into:
Frictional flows composed of a combination of grains and water in which the space between grains is filled by water. These flows are subdivided into:
- Hyperconcentrated density flows (sandy debris flows, some basal inverse grading, no normal grading, bedforms not preserved)
- Concentrated density flows (erosional base, scours & flutes, normal grading, lower bed massive with local inverse grading)
- Turbidity flows (subdivided on the basis of duration):
- Surges
- Surge-like flows (Bouma sequences)
- Quasisteady current
This classification scheme of sedimentary density flows assumes that any flow may change in type both down and across flow and with time at any one point. The strength and weakness of the classification is the spectrum of representative sediments involved, making it general, interpretative and qualitative.
Building from the concept of process driven interrelationships the interpretive models consideredcognize that there is a strong association between turbidites, mass transport debrites (MTD) and pelagic drape and/or condensed sequences. These models are used as a first pass guide to the character of the observations made in the field or lab. These models are then modified to meet the chf ones observations, rather than vice versa.
The turbidites & other frictional flows (Mulder & Alexander, 2001)
Hyperconcentrated density flows
The turbulent fluid motion of these hyperconcentrated flows is interpreted to have maintained high sediment concentrations of over 25% their volume, often in the form of sand and gravel sized particles. The fluid had no cohesion but grain-to-grain interaction supported and transported the sediment load. As the flow waned the sediment no longer supported and accumulated as sandy debris flows that are often sorted. These deposits show some basal inverse grading but no normal grading. Cross beds, and other bedforms, tend not to be preserved while composition varies between massive coarse silt, sand, and/or gravel.
Gravity drives these flows, whose velocity and character were a response to the steepness of the slope that the flow crossed. Unless the slopes were steep these flows had short transport distances and when the slope became gentler the sediment tended to freeze in place as grain-to-grain interaction took over. Hydroplaning may have prevented erosion.
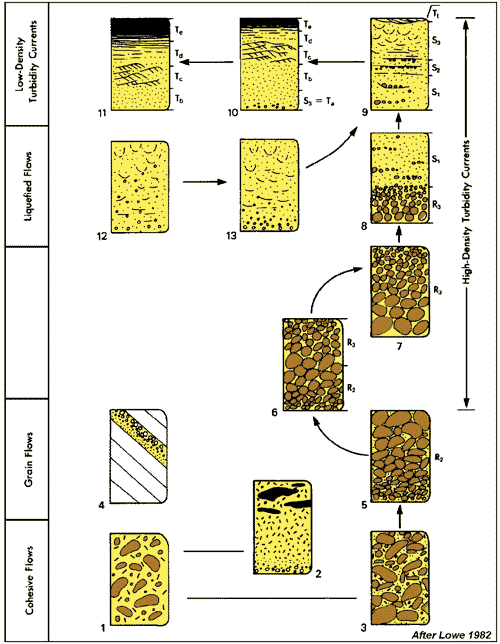
The deposits that Lowe, 1982 illustrates above as being deposited from high density turbidity currents match the Mulder & Alexander's (2001) concentrated density flow deposits explained below.
Concentrated density flow
These flows are defined on the basis of the turbulent fluid motion of the flow that supported from 10% to 25% sand sized particles. As this flow waned so the sandy debris flows illustrated above accumulated. The basal surface to these deposits is erosional and is often expressed as scours and flutes. These surfaces are evidence of rapid currents that moved down steep slopes both eroding and inhibiting the frictional interaction of grain-to-grain motion. So long as water was entrained between the grains the freezing of the sediment was prevented. As a slope decreased the sediment froze into place and the coarse bed load was deposited producing combinations of normal grading, and/or massive bedding with local inverse grading.
Turbidity flows
Even though they acknowledge that all natural sediment density flows are unsteady Mulder & Alexander (2001) subdivided these on the basis of duration of steady trubulent flow. These are:
- Short duration surges
- Surge-like flows in which the behaviour of the head of the flos the deposit (Bouma sequences or turbidites)
- Quasi-steady currents in which tour of the head of the flow is not as importthin the body of the flow
The turbidites
Few geologists have considered the term turbidite with as much attention to detail, or with as much enthusiasm, as Ganapathy Shanmugam. Whether geologists agree or disagree with Shanmugam’s (2000) poey still are likely confused as to how to proceed with classifying their own deepwater systems.
This web site uses the term turbidite as Kuenen (1957) first used it to represent a deposit from a turbidity current. This supports the published work of Kuenen’s student Bouma; the geologist who detailed the “man’s land” of the Annot Sandstone outcrops of SE France and established the first vertical facies model of turbidites (Bouma,1962). This stratigraphic section has become the type model for the "Bouma sequence”.
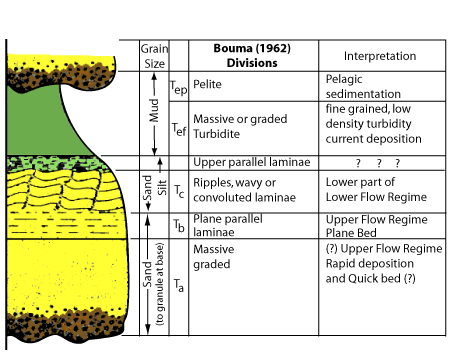
Since the Bouma paper of 1962 it has been demonstrated the turbidite deposit is a “member” of an evolutionary trend of vertically associated sediments that accumulate from debris flows, hyperconcentrated to concentrated density flows, and turbidity flows (Mulder & Alexander, 2001). As Parsons et al (2003) observe, “Gravity-driven motions produced from turbulent suspensions are an important sediment-transport process in the modern ocean and the deposits that result from them constitute a significant portion of the sedimentary record”.One of these deposits are turbidites. For instance on the continental margin of the Southwest Orphan basin in the Labrador Sea, sediment moves under gravity as cohesionless debris flows that are transformed basinward into fluids moving under gravity in the form of high-density turbidity currents (Tripsanas & Piper’s, 2006). In this latter case no hemipelagic deposits were found to separate the sand-gravel unit from the mud flow and the seismic data suggests that the mud-flow deposit was initiated by slope failure.
Mass-transport deposits (MTD) and debrites
Mass transport deposits have grouped as cohesive flows that include debris flows and clay-rich and silty mud flows by Mulder & Alexander (2001). Outcrops of these mass transport deposits record both subaerial and submarine depositional settings. Their downslope deepwater margin setting is described below.
One of the objectives of this web site is to help the user develop techniques to characterize potential and actual hydrocarbon reservoirs within these sediment complexes. Martinsen, and Posamentier, (2006) suggest to do this one should establish the deepwater stratigraphic architecture, its timing and tie to well and seismic data. Their basic approach examines age relationships, and characterizes the geometry, and the lithology of these sediments. They note that the processes that are responsible for the character of deepwater systems grade into one another as continuous spectrum down the subaqueous slope. These include
- Creep
- Sliding
- Slumping
- Debris flow
- Fall of material
All are controlled by fluid turbulence and gravitationally driven mechanisms.
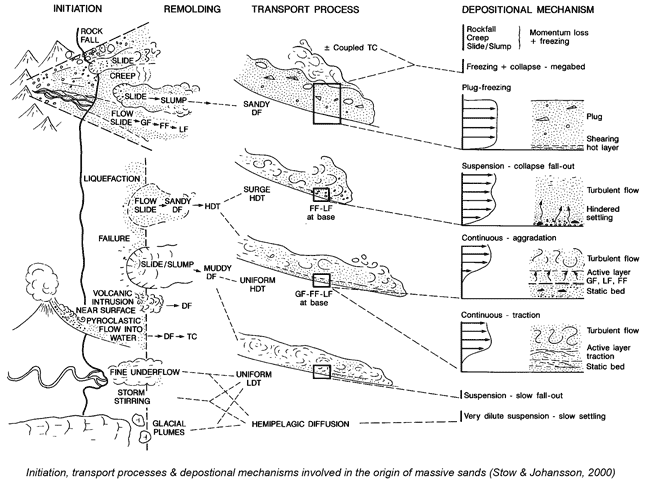
Nearly all-subaqueous slopes are unstable and down slope sediment movement is commonly expressed as mass transport complexes that vary in scale from cubic meters to several thousand cubic kilometers. Relative drops in sea level have often initiated large-scale margin collapse. This is often followed by confined chanalization down slope and then the cycle ends with unconfined fill that probably represents flushed shallow shelf sediments. Submarine fans in the Pyrenees (Pickering, and Bayliss, 2006) provide an example of this with Eocene mass-transport complexes (MTCs) confined to channels across them as they are transported to the basin-floor. Similarly on the Nile Fan, each of the large MTDs has a headwall scarp at the shelf edge or on the upper slope that forms a wide sloping corridor down which the sediment moves. “Channels systems appear to occupy these evacuation corridors immediately after most of these distinct mass failure episodes” (Newton et al 2006). Here and at other locations extensive deep-water mass transport deposits currently tend collect on slopes and basin settings. They form sheets, mounds lobes, and channel fills often 150 m or more in thickness (Posamentier & Martinsen, 2006). Their internal character includes heterogeneous deposits of mudstones to matrix-supported conglomerates with the chaotic, and slumped fabrics (Nilsen, et al, 2006).
Debris flows and leveed channels tend to build out from canyon mouths directly onto the basin plain. Currently in offshore West Africa, large Pleistocene aprons have formed with multiple leveed channel systems and associated debris flows. Avulsing channels and associated levees emerging from the canyon mouth occur and sidewall failures and rotational slips have produced debris flows in all stages of development (Sutton and Mitchum, 2006). Sheet deposits seaward and onlapping the apron deposits often show gravitational instability and a range of deformation processes. These form small-scale soft-sediment deformation structures (convolute lamination, dishes and pipes), intensely dewatered intervals and the development of low velocity slope ‘creep' bodies. These fabrics occur in the Early Permian deepwater deposits of the Tanqua area in South Africa (Wild et al, 2006).
The stacked deposits of co-genetic turbidity currents indicate other evidence of slope instability and debris flows are common to many down slope fan systems. These occur particularly downdip in relatively distal and lateral fan fringe settings, bypassing sandier mid-fan sections (Haughton et al, 2006). This sediment association is a response to erosion on unstable, tectonically controlled slopes or intrafan erosion during incision of the inner fan.
Posamentier and Martinsen (2006) indicate that mass transport units are commonly amalgamated. So much so that surfaces between successive mass transport deposits may be difficult to recognize. Never the less they identify two distinct mass transport end members: 1) those formed by coherent and commonly discrete blocks that moved along relatively smooth decollement surfaces, and, 2) those with a less coherent, disrupted fabric scoured into the lower surface with linear grooves that may diverge down-system. Blocks imbedded at the base of the flow mass and dragged across the underlying substrate form these groves. Posamentier and Martinsen (2006) intimate that the upper bounding surface of the latter often has irregular to hummocky relief, bounded laterally by steep to gentle flanks. In some instances the termini of the mass transport lobes exhibit extensive low-angle thrust faults associated with compression. This is supported by Butler et al’s, (2006) recognition that submarine mass transport complexes on the modern sea floor often display complex rugosity on their upper surfaces, defined by features interpreted to be rafted blocks, pressure ridges, detached faults and folds.
Pelagic drape
As authors that include Loutit & Kennett, (1981), Leckie et al (1990), Galloway (1989) and others have recognized, condensed sections accumulate as drapes of hemipelagic and pelagic mud rich in organic material over lowstand and
Figure showing how the lowstand systems tract autocyclic fan of a downslope basin floor fan can be enveloped by pelagic drape associated with a maximum flooding surface. Note the commonly autocyclic character of the cycles that terminate in shales and the transgressive surface over which a condensed sequence accumulates.
Unfortunately sedimentary drapes exist that have other origins than those associated with the maximum water depths of a sea level transgression and/or high stands. These other drapes are connected to autocyclic migration of the sand prone sections away from the axis of the deepwater fans, their channels, overbank fill and levees. For this reason the fine grained portions of homopycnal, hyperpycnal and hypopycnal sediment plumes (Bates, 1953); storm winnowed shelf sediment; glacially rafted material, pelagic fauna, and air born dust may dominate the depositional setting instead of the coarser potion of the fan driven deposition. From a stratigraphic perspective the fines that mark the passive phase of an autocyclic event look very similar in character to the fine-grained portion of the deepwater cycles and so be misinterpreted to be connected with the transgressive phase of a parasequence. However these autocyclic drapes are allostratigraphic in character and most have limited distribution within the internal variability of a fan or slump. Never the less, when they extend over a good portion of the fan complex, they can be used as stratigraphic markers. Even then, though the fine sediments match the pelagic drape described above, they often lack their condensed character and the associated microfaunas, glauconite, phosphorite and siderite, organic debris, and radioactive elements . Also because of their common lithologic character these may be mistakenly correlated with the other high frequency local events referred to above and without care their local character may be unrecognized. A mix of high frequency seismic tied to well data may help resolve this problem of miscorrelation.
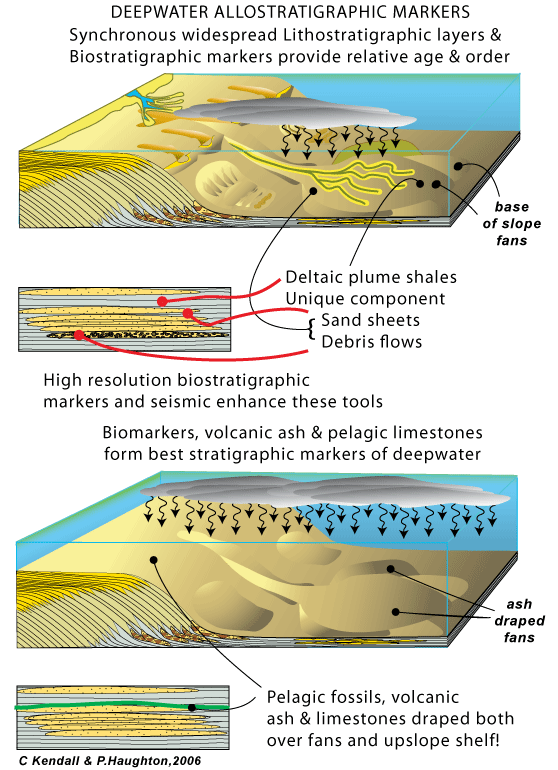
Click on highlighted Deepwater Gallery for access to a complete index to images, maps, diagrams and photographs of deepwater geology including the geology of Co Clare or access this gallery using the pull down menu on the header bar above.
Cited References
Bouma, A. H., 1962, "Sedimentology of some flysch deposits, a graphic approach to facies interpretation", pp 168; Amsterdam: Elsevier.
Butler, Rob, Adriana Del Pino Sanchez, Bill McCaffrey, Joris Eggenhuisen, Peter Haughton, Simon Barker, Bill Hakes, and Gillian Apps, 2006, "Dynamic formation of Rugosity on Mass Transport Complexes – Implications for Emplacement Dynamics" , SEPM Research Symposium: The Significance of Mass Transport Deposits in Deepwater Environments II, AAPG Annual Convention, April 9-12, 2006 Technical Program
Dott. R.H., JR., and Bourgeois, J., 1983, "Hummocky stratification: significance of its variable bedding sequences": reply to discussion by R.G. Walker et al.: Geological Society of America, Bulletin, v. 94, p. 1245–1251.
Csato, I., C. G. St. C. Kendall, 2001, "Modeling of stratigraphic architectural patterns in extensional settings – Toward a conceptual model", Computers and Geosciences.
Dott and Bourgeois (1983)
Galloway, W.E., 1989. "Genetic stratigraphic sequences in basin analysis". I. Architecture and genesis of flooding-surface bounded depositional units.
Haughton, Peter, Chris Davis, and William McCaffrey, 2006, "Facies Prediction in turbidite Fan Systems – Nature and Significance of ‘Linked Debrites' in Sand-Rich Versus Mixed Sand-Mud Systems" Recent Advances in Siliciclastic Facies Models: Implications for Reservoir Characterization II (SEPM), AAPG Annual Convention, April 9-12, 2006 Technical Program
Kuenen, Ph H, 1957, "Sole markings of graded graywacke beds", Journal of Geology, 54, 120-147
Leckie, D.A., Singh, C., Goodarzi, F., and Wall, J.H., 1990, "Organic-rich, radioactive marine shale: a case study of a shallow-water condensed section, Cretaceous Shaftesbury formation, Alberta, Canada": Journal of Sedimentary Petrology, v. 60, p. 101-117.
Loutit, Tom S., and James P. Kennett, (1981), "New Zealand and Australian Cenozoic Sedimentary cycles and Global Sea-Level Changes", Bull Am Association of Petroleum Geologists, Vol. 65 Pages 1586 – 1601
Lowe, D.R. (1982) "Sediment gravity flows: II. Depositional models with special reference to the deposits of high-density turbidity currents". J. Sed. Petrol., 52, 279-297.
Martinsen, Ole, and Henry W. Posamentier, 2006, “The Character and Genesis of Mass Transport Complexes II: Processes, Classification and Insights from Outcrops”, Recent Advances in Deepwater and Shelf Siliciclastic Facies Models: Implications for Reservoir Characterization (SEPM), AAPG Annual Convention, April 9-12, 2006 Technical Program
Miall, Andrew D. 1999, In Defense of Facies Classifications and Models, Journal of Sedimentary Research, Vol. 69, No. 1, January, 1999, P. 2–5
Mulder, Thierry and Jan Alexander; 2001, “The physical character of subaqueous sedimentary density flows and their deposits”; Sedimentology: 48, 269-299
Nilson, C. Hans, Carlota Escutia, and John E. Damuth, 2006, "Variation of Mass-Transport and turbidite Deposits in Different Continental Margin Settings and Time Periods", SEPM Research Symposium: The Significance of Mass Transport Deposits in Deepwater Environments AAPG Annual Convention, April 9-12, 2006 Technical Program
Newton, C. Simon, R. Craig Shipp, Ahmed Farouk, Erik D. Scott, and Timothy M. Farnham, 2006, "Significance of Large Quaternary Mass Transport Deposits on Exploration and Development of the Nile Fan, Offshore Egypt" SEPM Research Symposium: The Significance of Mass Transport Deposits in Deepwater Environments AAPG Annual Convention, April 9-12, 2006 Technical Program
Nilsen, Tor H., Gary S. Steffens, and Joseph J. R. Studlick, 2006, "Mass Transport Deposits in Deepwater Outcrops: Depositional Setting(s), Types, and Recognition", SEPM Research Symposium: The Significance of Mass Transport Deposits in Deepwater Environments II, AAPG Annual Convention, April 9-12, 2006 Technical Program
Parsons, Jeffrey D., William J. Schweller, Charles W. Stelting, John B. Southard, William J. Lyons, and John P. Grotzinger, 2003, "A Preliminary Experimental Study of turbidite Fan Deposits": Reply: Journal of Sedimentary Research,2003 73: 839-841
Pickering, Kevin, and Nicole Bayliss, 2006, "Mass-Transport Complexes (MTCs), and Tectonic Control on Confined basin-Floor Submarine Fans, Middle Eocene, South Spanish Pyrenees", SEPM Research Symposium: The Significance of Mass Transport Deposits in Deepwater Environments II, AAPG Annual Convention, April 9-12, 2006 Technical Program
Posamentier, Henry, and Ole Martinsen, 2006, "The Character and Genesis of Mass Transport Complexes I: Geomorphology and Process Sedimentology from 3-D Seismic Data", SEPM Research Symposium: The Significance of Mass Transport Deposits in Deepwater Environments, AAPG Annual Convention, April 9-12, 2006 Technical Program
Pyles, David, and Renaud Bouroullec, 2006 “Processes and Facies Associations in basin-Margin strata of Structurally Confined Submarine Fans: Example from the Carboniferous Ross Sandstone, Ireland”, Recent Advances in Deepwater and Shelf Siliciclastic Facies Models: Implications for Reservoir Characterization (SEPM) AAPG Annual Convention, April 9-12, 2006 Technical Program
Shanmugam, G., 2003, "A preliminary experimental study of turbidite fan deposits":Discussion: Journal of Sedimentary Research, v. 73, p. 838-839.
Sutton, Jason P., and Robert M. Mitchum, 2006 “Deepwater West Africa Waterbottom Debris Flows Captured and Analyzed by 3-D Visualization Techniques” SEPM Research Symposium: The Significance of Mass Transport Deposits in Deepwater Environments I AAPG Annual Convention, April 9-12, 2006 Technical Program
Tripsanas, Efthymios K. and David J.W. Piper 2006, "formation of Cohesionless Debris Flows and turbidity currents from Sediment Failures: A Case Study from the Continental Margin of Southwest Orphan basin, Labrador Sea", SEPM Research Symposium: The Significance of Mass Transport Deposits in Deepwater Environments II, AAPG Annual Convention, April 9-12, 2006 Technical Program
Wild, Richard, David Hodgson, Stephen Flint, and Willem Van der Merve, 2006, “High-Resolution stratigraphy of Multiple, Vertically Stacked Slope Channel Complexes, Tanqua Depocentre, Karoo basin, South Africa”, Recent Advances in Deepwater and Shelf Siliciclastic Facies Models: Implications for Reservoir Characterization (SEPM) AAPG Annual Convention, April 9-12, 2006 Technical Program